Article: The Urgency of Embodied Carbon and What You Can Do about It
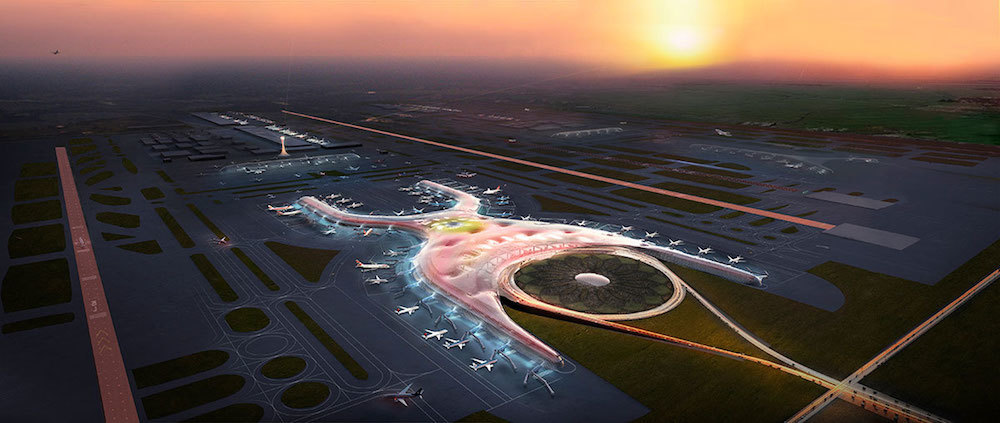
Article originally posted on Building Green website here.
This article does an excellent job laying out embodied energy in building materials. The most important piece of the embodied energy puzzle whether working with concrete, steel or wood is to "use only what you need". Below are a few excerpts from the article but you can read the full article here.
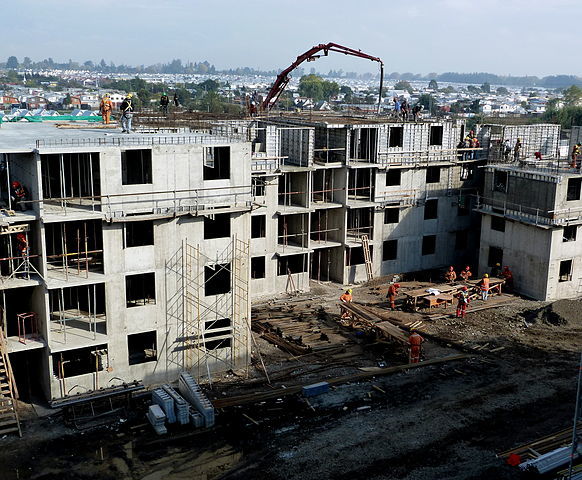
Concrete and cement
Concrete has a large footprint because of the carbon-emitting process used to make one of its most important ingredients—the binder portland cement (see Reducing Environmental Impacts of Cement and Concrete). By some estimates, production of portland cement is responsible for 5% of total global CO2 emissions. Replacing some cement with supplemental cementitious materials (SCMs) like fly ash or blast-furnace slag is a go-to way for project teams to reduce the embodied carbon of the concrete in their projects.
But that’s not always as simple as it might sound, and structural engineers have some advice about how to do it right.
Engineering firm Walter P Moore has conducted about 20 whole-building LCAs in pursuit of the Building Life-Cycle Impact Reduction credit under LEED version 4, according to Dirk Kestner, P.E., director of sustainable design. Kestner’s takeaway? Every project—even those with wood structural systems—contains substantial amounts of concrete, and cement content is one of the largest contributors to embodied carbon on a project.
Steel
By weight, steel has a much higher embodied carbon footprint than concrete does—with one ton of steel representing approximately a ton of greenhouse gas emissions. According to the World Steel Association, steel production is responsible for 6.6% of greenhouse gas emissions globally—more than portland cement (see Better Steel, Lower Impacts). Those global numbers reflect use of dirtier technology in much of the world, which is still using basic oxygen furnaces (BOF) rather than electric arc furnaces (EAF). In North America, the industry has mostly switched over to EAF technology—the process used to recycle steel. This, along with a cleaner electrical grid, has resulted in a 36% reduction in the industry’s carbon footprint since 1990, according to Mark Thimons, P.E., vice president, sustainability, at the Steel Market Development Institute.
So that’s the first rule of thumb for reducing the embodied carbon of steel on a project: specify steel produced in North America—or, if that’s not possible, at least specify recycled steel, which uses the better EAF technology.
The only other real option for reducing steel’s footprint is to use less—a practice that’s even promoted by the Steel Market Development Institute, a trade group. Aside from choosing North American steel, “the other advice that we always give to architects and especially engineers is just to be as efficient as possible in designs,” Thimons told BuildingGreen. “We really encourage the concept of working with an integrative process. It can result in some of those kinds of savings; more efficient designs result in better and lower environmental footprints.”
Structural engineer Mark Webster agrees, advocating for “approaches like composite design, where the steel and concrete slab work together and can reduce the size of the beams.” He added that “choice of lateral system can have a big impact on the quantity of steel” as well. Braced frames with diagonal braces use far less steel than moment frames, for example. “You end up with a lot more steel using those moment-resisting frames,” he said. “For architects, it’s nice to use moment frames because you don’t have diagonal braces,” but braced frames can be strategically designed to reduce the architectural impact. That’s a good reason to get the structural engineer involved early when looking to reduce embodied carbon.
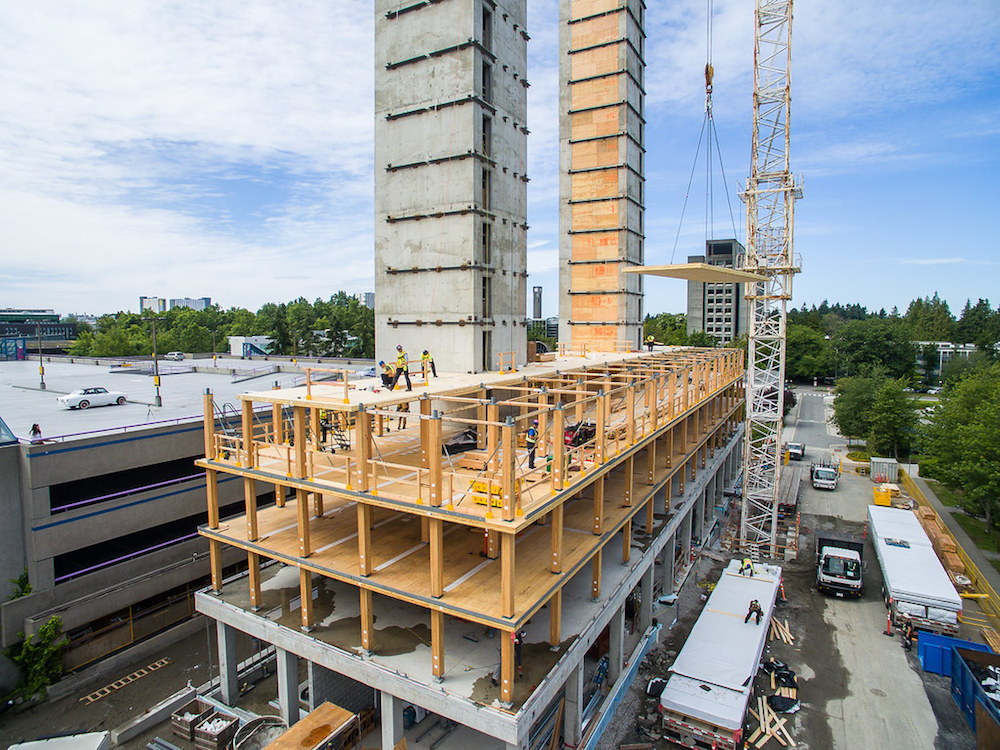
Structural wood
You may have heard (including from BuildingGreen) that building with wood instead of concrete or steel has major carbon benefits. It seems to make sense, since wood products sequester carbon, while concrete and steel are made by burning fossil fuels. Interest in building with mass timber structural products like cross-laminated timber (CLT) has skyrocketed, in part because of the presumed lower embodied carbon impacts.
But a few scientists are asking everyone to slow down, contending that LCAs have grossly overestimated the benefits of wood.
“Wood is very tricky right now,” said Stephanie Carlisle, principal at KieranTimberlake and the lead developer of the Tally whole-building LCA software tool. “There is a big debate happening.” And that’s frustrating for designers who want guidance they can use.
“The more we’ve dug, the more [the numbers] seem to be all over the place,” said Arup’s Yang. “There is so much uncertainty carried with them.”
This uncertainty has many sources.
First of all, LCAs mostly give wood a free pass when it comes to the state of the forest after harvesting. But a lot of carbon in forests is stored in the soil and below it, and it’s unclear how much carbon and methane (a more potent greenhouse gas) is released when harvesting … and how much that depends on how the wood is harvested.